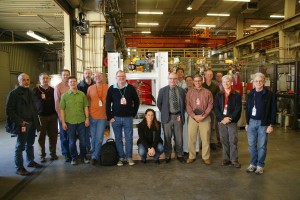
Happy scientists at the end of acceptance-testing a new X-band klystron at SLAC in January. The testing team consists of people from CERN, SLAC, PSI, Trieste and the klystron manufacturer CPI. Image: SLAC
The planned CLIC accelerator would use a unique way of accelerating its electrons and their anti-particles, positrons: two accelerators would sit side by side, one, the main linear accelerator or “linac”, to get the beams of particles from source to collision, and the other, the “drive beam”, to pass as much power as possible on to the main beams. This gives them a big push, it increases the rate at which they accelerate – their gradient. The CLIC main beam will have an acceleration gradient of 100 Megavolts per metre. For comparison: the ILC gradient is 31.5 Megavolts per metre (MV/m); the LHC accelerates at 5 MV/m, but as it is a storage ring, the beam can pass through the accelerating structures several times, while beams in a linear accelerator have only one shot at being accelerated before they collide.
In order to test the accelerating structures, CLIC scientists build test stands that are not powered by the CLIC drive beam but by power sources called klystrons that provide radiofrequency power in the X-band range. They believe that these klystron test stands (combined with high-gradient accelerating structures) could be useful for future free-electron lasers, special accelerator-driven lasers that provide very particular laser light for studying materials, biological samples, molecular processes and much more. “The high gradient means that the accelerator can be very short because beams reach the designated energy much more efficiently,” explains Walter Wuensch, head of the X-band R&D for CLIC. “We have done a lot of research on getting the gradient for CLIC, we have a lot of experience with X-band systems and sources are now available commercially. All this makes an X-band accelerator comparatively affordable.” This means labs or companies can build or upgrade free-electron lasers and make them available for all sorts of applications.
Wuensch says that both the technology and beam diagnostic tools have been tested to the core. “We are confident we can build linear accelerators for free-electron lasers according to the desired specifications,” he says. During a High-Gradient Day at last week’s CLIC workshop, several light source operators from Switzerland, Turkey, Italy, China, Australia and Sweden exchanged their specs, wishes and future plans with the CLIC team. A free-electron laser driven by CLIC technology would be a dream come true for Wuensch and his colleagues.
Another topic at the High Gradient Day was the involvement of CLIC expertise medical projects. One of these, TERA TULIP, looks into operating a proton accelerator for cancer therapy. CLIC’s high-gradient experience could help make the gantry through which the beam is passed to the patient shorter and lighter by installing the accelerator on the gantry itself, thus reducing the number of bending magnets needed for the proton beam and making the gantry more compact. If it could move around the patient and provide high-precision beams, damage to non-cancerous tissue could be avoided.
A few other potential applications which might benefit from X-band and high-gradient technology were discussed at the industry day, “but these are further down the line,” says Wuensch. We’ll make sure to let you know when the first free-electron laser using CLIC technology comes online.
A version of this story first appeared on the CERN homepage.
Recent Comments