Lately, scientific communities are in need of higher-energy light particles.
To do this, scientists are extending the existing technology of undulator magnets, or undulators. The device uses magnets to wiggle a particle beam into giving up light, which can then be used for a whole host of scientific applications.
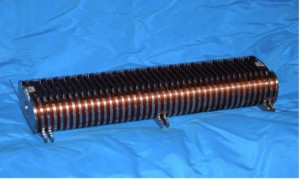
An early, 42-magnetic-pole test coil of Argonne National Laboratory's planar superconductor undulator. A planar undulator has two cores, each wound with superconducting wire, which are placed on opposite sides of the electron beam. The electron wiggles will lie in a plane, and the resulting photons will be linearly polarised. Image: Yury Ivanyushenkov
“Undulators are specialised beasts,” said Elizabeth Moog, head of the Magnetic Devices Group at Argonne National Laboratory. Different applications require different characteristics in their light.
Argonne in the US and Daresbury and Rutherford-Appleton laboratories in the UK are developing superconducting undulators to meet both high-energy physics and light source scientific communities’ various photon needs.
One goal of the two UK labs is to help fulfil the ILC’s need for a positron source. Jim Clarke, recent Positron Technical Area Group Leader for the Global Design Effort, oversees undulator work at the UK labs. Last year his group successfully produced two 1.75-metre undulator prototypes.
Argonne has also developed an undulator prototype for other science programmes. Theirs is the first real planar superconducting undulator being built for installation in the United States.
The name of the technology relates its modus operandi: it causes an otherwise straight-shooting particle beam to undulate.
A row of magnets of alternating polarity manoeuvres an electron beam to wiggle as it travels down the axis of the magnet row. With every turn in its sinusoidal path, the electron beam radiates light particles, or photons.
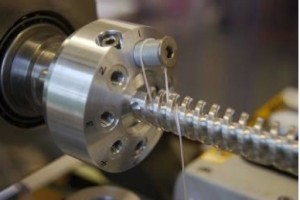
Daresbury and Rutherford-Appleton laboratories' helical superconducting undulator at the beginning of a winding. Wires arranged are around the beam tube like stripes on a barber pole. This allows the photons to be circularly polarised, which can then produce circularly polarised positrons. Image: Jim Clarke
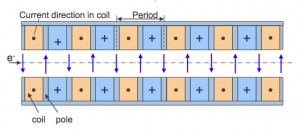
Diagram of an undulator cross-section. An undulator period is measured from one winding to the next of the same polarity. An electron beam passes down the axis of the series of magnets, undulating as a result of the alternating polarities. The direction of the arrows in the undulator gap show the direction of the magnetic field seen by the electrons. Image: Yury Ivanyushenkov
In the ILC plan, the radiated photons are directed at a metal target, and the resulting collision produces positrons. The process calls for 10-megaelectronvolt photons. In 2006, when the UK labs began collaborating on the project, photon production of that energy was out of reach.
“Nobody had looked into whether that was practical or not,” said Clarke. “No magnets with our parameters had been made before.”
One difficulty lay in squeezing enough magnetic field into a short enough distance to achieve the required photon wavelength for a particular beam energy and geometry.
The same challenge faced researchers on the other side of the Atlantic at Argonne. They’d been developing undulators for their Advanced Photon Source (APS), a synchrotron light source that provides X-ray beams to hundreds of scientists in fields as diverse as biology, geology and materials science. Argonne’s undulators are designed to produce photons in a variety of wavelength ranges; the superconducting undulator is being designed to produce 20- to 25-kiloelectronvolt photons.
Traditional undulators have made use of permanent magnets. DESY and the European XFEL use them for their undulators. But for many of the newer science projects, those magnets wouldn’t pass muster since the fields of permanent magnets are only so strong and so uniform.
Superconducting electromagnets, on the other hand, can pack a good deal more magnetic strength into a few millimetres of undulator. The greater magnet strength leads to higher-energy photons.
Superconducting undulator magnets are constructed by winding superconducting wire around a core. The electron beam travels nearby. The greater the current in the wire, the stronger the resulting magnetic field seen by the electron beam.
“We’ve been able to produce the best-looking magnetic field of any undulator I’ve seen,” said Moog.
Increasing the electron beam energy does have a cost, so scientists also try to draw out higher-energy photons by doing something less expensive – reducing the so-called period length. The period length is the distance from one turn of wire to the next. Shrinking that distance bolsters the photons’ energy.
After four years, the Daresbury-Rutherford-Appleton team has been able to shrink what was originally a 14-millimetre period to one only 11.5 millimetres long, the desired period length for producing the ILC’s 10-MeV photons. In Argonne’s prototype, the team has chosen a 16-millimetre period length to meet the requirements of APS users. The standard APS permanent-magnet undulator has a period length of 33 millimetres.
Even the simple act of winding comes with its own set of complications. Both Argonne and the UK scientists have tested their undulator using niobium-titanium wire, a tried-and-true material for the superconducting magnets. But another material, niobium-tin, has the potential to kick up the critical current even higher. The problem is that the standard insulation doesn’t survive the high-temperature treatment process required to make it superconducting in the first place. Researchers are continuing tests to determine whether niobium-tin is a viable superconductor option for this application.
“Though the magnet design is quite simple, realising it in practice is very difficult,” said Clarke.
Daresbury and Rutherford-Appleton labs have now produced a working, full-scale model capable of generating their target photon energy and Argonne hopes to follow suit in a couple of years.
“It’s such interesting technology both for the ILC and for so many other applications,” said Yury Ivanyushenkov, technical leader for the superconducting undulator at Argonne. “It’s promising, and once the technology is well established, many people, we believe, will be interested.”
Recent Comments