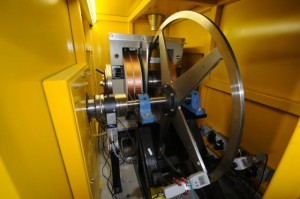
The rotating positron target sits in a protective steel bunker. A dipole magnet (rear) creates a one-tesla magnetic field to help with tests related to eddy currents. The two light grey boxes in the foreground measure the wheel rim’s temperature. Image courtesy of Ian Bailey.
The ILC’s positron target is about to undergo tests of fortitude.
Researchers with the Global Design Effort are developing a positron target for the ILC that can stay cool while producing about 1014 positrons per second for collisions with electrons. To accomplish that, it would have to withstand a barrage of photons, stave off feverish heat, and do it all while spinning stably at 2,000 revolutions per minute.
“We’re trying to do something no one’s done before, and we’re on track,” said Ian Bailey of the Cockcroft Institute in the UK.
The heart of the ILC positron source is a one-metre-diameter, five-spoke titanium alloy wheel. A beam of ten-megaelectronvolt photons strikes the wheel’s rim, the positron target, as it spins. The overall impact of the photon beam is thus spread around the target, helping to mitigate the damage that would occur if the particle onslaught were concentrated on a single spot. Photons striking the target create electromagnetic showers in which positrons are born.
Scientists completed a prototype of the wheel at Cockcroft and at Daresbury Laboratory in the UK in 2008. Tests have shown that it should be able to survive not only a photon fusillade, but also overwhelming heat.
“The photon beam that’s hitting the target is intense,” said Jim Clarke of Daresbury Lab, who, as group leader of the GDE Positron Technical Area Group, headed the effort to construct the hardy wheel.
The photon radiation is one source of heat. Yet another arises thanks to a device that’s positioned directly behind the target, the flux-concentrating magnet.
An integral part of the ILC positron source, the flux-concentrating magnet captures positrons coming off the target and steers them on the path toward the accelerating cavities. Unfortunately, the magnetic fields near the spinning wheel also create so-called eddy currents within the metal. Those currents, in turn, kick up its temperature.
Modelling eddy currents is a thorny business. Three different teams simulating the problem produced three very different responses to it. With an additional 20 kilowatts of heat potentially circulating the wheel, it was worrisome.
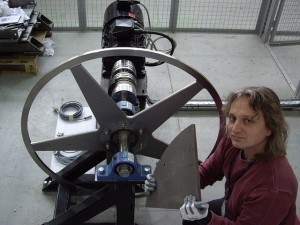
Ian Bailey holds one of the wheel’s off-cuts next to the rotating positron target prototype. Off-cuts are used for wheel material studies. A motor sits behind the wheel.
“A rotating wheel in a magnetic field is a nineteenth-century physics problem,” Bailey said. “It doesn’t sound cutting edge, but it isn’t as simple as physicists think it’s going to be when they first look at it.” So the team took to the problem by actually constructing a target, then comparing it with various models.
“It turned out the best way to get the right model was just to build a wheel, put it in a magnetic field and turn it,” said Clarke. Tests show that the current target prototype should handily overcome any adverse effects from the eddy currents. But the team will continue comparing experiment against models to reconcile the two.
They will also aggressively combat the unavoidable heat by sending cold water through the target’s spokes and around its rim, where the photons strike.
“To get the water in the wheel and out again is quite complicated,” said Clarke. Now a team at Lawrence Livermore National Laboratory in the US will test a mockup of the target, in part to make sure the wheel’s shaft can handle the cooling water flow.
Heat is not the only peril of rotation – rotation itself can create its own dangers.
“You could be nervous that it could come off its bearing and fly across the building,” Clarke said, which is why the target is kept under a steel bunker with extra protection. And while it is assuring to know the bunker will protect it, Daresbury Lab tests demonstrate the fast-moving wheel won’t fly off the handle under the force of its own spin.
Future tests at Livermore will also vet the system’s mechanical stability.
“We’re trying to build exactly what we would build for the real experiment,” said Livermore’s Jeff Gronberg. They’ll examine the rotordynamics of the support system to make sure it hits no resonant frequencies, which could cause undesirable vibrations.
Studies of the rotordynamics must also include tests on vacuum seals. The wheel is situated on a shaft that passes from vacuum into air. Even as the shaft spins at 2,000 turns per minute, the material that seals the space where the rotating shaft makes contact with the vacuum chamber wall has to hold. That isn’t trivial.
The Livermore team is currently inspecting a ferrofluidic seal, which should allow the shaft to spin freely even as it keeps the air out.
The Livermore mockup target system will run continuously for six months. It may seem like a long time to be running 24-7, but Gronberg reminds us of the real-life target stamina that’s called for in the actual ILC.
“We’ll never be running the main accelerator with this wheel not running,” he said.
“The positron target is a complicated engineering object,” Bailey said. “The ILC is the most challenging positron source project out there, that I’m aware of. But so far, the baseline’s looking good.”
Recent Comments