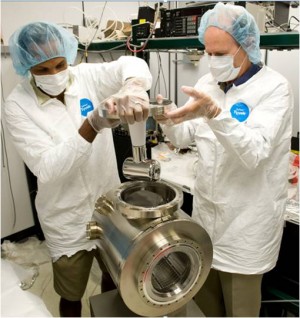
Suspended from the white ceramic insulator, the cathode electrode is lowered into the high-voltage chamber by Phil Adderley and Jim Clark, members of Jefferson Lab's Electron Gun Group, during assembly of an ILC-like prototype spin-polarised electron photogun. This photogun was later installed at Jefferson Lab's Continuous Electron Beam Accelerator Facility and successfully operated for their nuclear physics programme. Image: Jefferson Lab
Opposites attract and like charges repel. This simple phenomenon explains why electron beams spread out over space and time, much to the dismay of physicists who need tight particle beams for collision experiments.
To counter this phenomenon, known as space charge-induced emittance growth, the electron gun group at Jefferson Lab in the US is pushing the limits of high-voltage electron guns with new and improved designs.
This work is motivated and funded by the International Linear Collider R&D programme, the goal of which is to develop a 140-kilovolt (kV) electron gun to produce spin-polarised electron beams.
“The more energy you give the electron beam from the start, the stiffer the beam becomes,” said Matt Poelker, leader of Jefferson Lab’s Electron Gun Group. “The space charge forces that frustrate us get smaller and the beam is much more manageable.”
Electron guns are electrical components that produce electron beams. They can be found in devices ranging from televisions to electron microscopes and particle accelerators.
An electron’s spin is a quantum-mechanical property describing its intrinsic angular momentum. Spin-polarised electron beams are made up of electrons with uniformly aligned spins. They provide an additional tool in physicists’ toolboxes to help probe what goes on inside the nucleus, Poelker said.
Polarised electron sources have four components: a gallium-arsenide photocathode, high voltage, a laser and a gun vacuum assembly.
A photocathode generates spin-polarised electrons upon laser-excitation with the appropriate wavelength of light. Once they leave the photocathode, at higher voltages, electrons are accelerated to higher energy and faster speed, making them travel together more tightly. But as with most things in life, this advantage comes with a trade-off: higher levels of field emission, the uncontrolled liberation of electrons from metallic surfaces near the photocathode.
“Those electrons don’t go where you want them to go,” Poelker said. “They degrade the vacuum in the gun chamber, and worst case, they might actually punch a hole in your gun chamber.”
The source of field emission is the cathode electrode, a metal structure that holds the photocathode inside the gun and provides electrostatic focusing of the beam. By employing a compact ceramic insulator that extends into the high-voltage chamber, the team reduced the amount of metal that could contribute to field emission, said Joseph Grames, deputy head of Jefferson Lab’s Electron Gun Group.
While the previous gun design operated well up to 100 kV, two newer designs are pushing the limits: Jefferson Lab’s CEBAF accelerator currently operates an electron gun at 130 kV, and a Jefferson Lab test facility operates another gun at 225 kV with no observable field emission.
The goal now is to further optimise the designs and test them at even higher voltages – up to 350 kV.
“My hope is that we can achieve this 350-kV bias voltage goal relatively soon so that it can be included in the Technical Design Report that will get written up before the ILC R&D programme comes to a conclusion,” Poelker said.
The ILC would collide a spin-polarised electron beam with a spin-polarised positron beam. While initial Jefferson Lab efforts focused on the development of high-voltage electron beams, more recent efforts have shifted toward making polarised positrons.
The traditional approach to generating positrons is to send a very high-energy electron beam into a tungsten target. The resulting photons can convert into electron-positron pairs. The limitations of this technique are that it requires a large accelerator and provides unpolarised positrons. The ILC has evaluated alternative approaches, such as helical undulators and Compton backscattering, which still require the high-energy accelerator but will produce polarised positrons.
As an alternative approach, the Jefferson Lab group plans to shoot relatively low-energy spin-polarised electrons at a millimetre-thick piece of tungsten foil, collect the resulting spray of positrons, and measure the fraction of polarisation that is transferred from the electron beam, Grames said.
“You don’t create as many positrons with this method but it doesn’t require a big accelerator,” he said. The method is now being explored largely because of relatively recent advances in spin-polarised electron sources, which have demonstrated sustained milliamp beam intensity.
While this work is motivated and funded by the ILC R&D programme, Poelker said it has greatly benefitted Jefferson Lab’s other research areas, including its nuclear physics programme.
“What we’re able to do now, not too long ago we thought was crazy,” said Grames, who got his Ph.D. in the late ‘90s, nearly two decades after the first spin-polarised electrons were injected into a high-energy accelerator at SLAC. “It has been very rewarding to contribute to developments over the last ten years as a scientist and observe the excitement it generates for ongoing experiments and the potential for future facilities.”
To learn more, see these two papers, which describe the electron gun:
Recent Comments