Some physicists joke about electrons, saying that you cannot collimate them, you can only make them angry.
Collimation is the removal of low-intensity, high-amplitude particles from a particle beam, typically by absorption into a metal. These unwanted particles, which make up what’s known as the halo, could cause high backgrounds in collision data and wreak havoc on detector components.
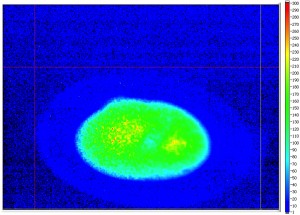
Figure 1. Lower-dynamic-range transverse beam profile measured in Jefferson Lab’s free-electron laser injector. The blue halo intensity is about 300 (arbitrary units) less than that of the green core. Image courtesy of Pavel Evtushenko
Pavel Evtushenko, physicist at Jefferson Lab in the US, hopes his work will help create clean, tight electron beams for future electron-positron collision experiments such as those at the ILC. He and his colleagues in the laboratory’s Free Electron Laser Division are developing tools to perform improved beam diagnostics and beam dynamics studies for high-current electron linacs.
This year Evtushenko was awarded the US Department of Energy Office of Science Early Career Research Award to develop high-dynamic-range diagnostics for linear particle accelerators.
“Pavel’s been one of our brightest young scientists,” said George Neil, who leads the Free Electron Laser Division. About seven years ago, Neil met Evtushenko in Germany. “As soon as I met him, I said, ‘I’ve got to have that guy here at Jefferson Lab.’ So I began my not-so-subtle arm twisting to convince him to come over and join our group.”
With his recent award, Evtushenko will spend the next five or so years developing experiments to better diagnose the conditions that form halos around electron beams.
Not all particle beam halos are created equal. Gaussian proton beams, such as those at the Tevatron and LHC, are characterised by halos that reside around the edges and are typically removed by placing in the beam’s path tungsten blocks, which absorb protons in the halo.
Electron beam profiles, on the other hand, are highly variable and poorly defined as halo particles reside throughout the beam and are more difficult to remove. When electrons strike a collimator, instead of being absorbed, they can be scattered back into the beam with altered trajectories, so creating an irritating new halo. Good-humoured physicists often refer to this as the electrons getting angry.
“It’s a joke, but there’s some truth in it,” Evtushenko said.
The three-pronged goal of Evtushenko’s research is to develop a high-dynamic-range technique to measure the profile of an electron beam, use the technique to study beam dynamics and evolution over space and time, and develop solutions for manipulating and controlling the beam, including removing the pesky halo.
The dynamic range of an instrument is the ratio between the largest and smallest values it can measure. For example, a digital camera has a dynamic range equivalent to the ratio between the largest and smallest measurable light intensities it can capture. With a high-dynamic-range camera, a photographer can take a picture of a sunset, capturing the bright sun and the shadows on the water in a single shot.
Measuring the profile of an electron beam is like taking a picture of the beam’s cross-sectional area. An electron beam profile has peaks and valleys, which, by current methods, can be measured with diagnostics with a dynamic range of 1,000.
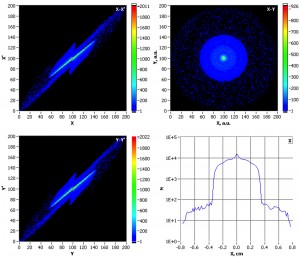
Figure 2. The same beam profile as Figure 1, this time from numerical simulations. Upper right: Transverse beam profile. Upper left, lower left: Transverse beam phase space distribution, demonstrating the development, due to the nonlinear beam dynamics, of the halo. Image courtesy of Pavel Evtushenko
With today’s ever-increasingly powerful computers, researchers simulate electron beams of millions of particles, including those that make up the halo. To test the reliability of these simulations, Evtushenko would like to match the computers’ kind of dynamic range – a range of about 1,000,000 – in beam diagnostics instruments. If tests using high-dynamic-range diagnostics bear out the high-precision simulation results, then scientists can use computers to do many of the studies rather than going into the lab to make time-consuming measurements.
Evtushenko’s proposal involves upping the dynamic range through a variety of techniques. One is to use so-called laser wire to get a better picture of the beam’s longitudinal shape. Both of Jefferson Lab’s accelerators, the CEBAF and the accelerator that powers the free-electron laser, generate continuous-wave electron beams at very high repetition rates, in contrast with a beam with more separated bunches. It affords researchers the opportunity to use ultra-short laser pulses, or laser wire, to measure the electron beam profile. Higher average current and high repetition rate ease the task of making longitudinal phase space measurements of the beam and enable higher dynamic range measurements.
Another technique is to use an imaging method similar to that used in astronomy, where different celestial bodies and lights are imaged with different exposure times, all of which are later pieced together to form a clearly rendered whole. With a zipping particle beam, of course, one can’t take much liberty with time, so the challenge is to quickly image different parts of the particle beam using different gains suited to the part of the beam being imaged. An algorithm helps put together the high-dynamic-range profile.
Trying to get that kind of clarity with frenetic, angry electron beams won’t be simple. “But I guess if any of this were easy, it would have been done already,” Evtushenko said.
It’s worth the trouble for cleaner beams.
“If we can learn to predict the sources of these small parts of the beam outside the bulk and then control or reduce the level, we can make more powerful beams that won’t destroy themselves,” Neil said.
Evtushenko plans to use the technique he develops to capture an image of the low-energy beam shortly after it emerges into the accelerating cavity.
“It’s very encouraging to get approval for this work,” Evtushenko said, referring to the DOE award. He’s happy to be involved in work that will help bring about the next generation of light sources, he said. And, he hopes he can keep the electrons happy, too.
Recent Comments