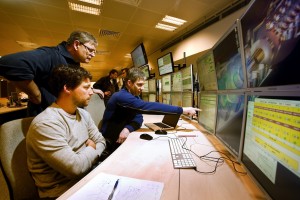
Julien Branlard, Nick Walker and John Carwardine discussing settings for the long bunch train studies in the accelerator control room at DESY. Image: Thies Rätzke
Accelerator theory and operating experience are all well and good. But if you’re planning and researching the next-generation particle accelerator, you sometimes need to crank things to their limits in order to get a step further. The 9-mA team has descended again on DESY’s FLASH accelerator in March to share control room space with the DESY operators, turn knobs and run programs that bring the 200-metre superconducting linac somewhat closer to how the ILC would run, delivering a wealth of data to the team and producing some very interesting results for FLASH operation as well.
The overall goal of the 9-mA studies is to run FLASH with long bunch trains and heavy beam loading, with cavities at the very top of their possible gradients and, accordingly, energy gains.
Tuning for maximum performance is an intricate interplay of information, with signals being fed back and forward to the knobs on each of the individual cavities so that all of them work together to reach the goal. It’s no use if one of them runs off with a high gradient only to lose it again after a split second or, worse, quench and cause the whole chain to break down.
For the ILC to reach its intended performance, all the cavity gradients should be as flat as possible – meaning constant and stable – during the 800-microsecond beam pulse. If they change as a result of accelerating a train of bunches, some of the cavities could quench, forcing operators to run them at lower voltages and hence energy gain. During the studies in March, the team had set itself a goal of keeping gradients flat to within 1%: they reached 0.2% – much better than required.
“It’s all done by software that manipulates each cavity’s individual setup, tailoring it to its optimal individual gradient and to the overall operating beam current,” says study leader John Carwardine from Argonne National Laboratory, US. “First you calculate the optimal theoretical settings for the knobs we have (piezo tuners and power couplers), and with those you can get very close, but to really nail it the automated program found the right position by an iterative approach.” Meaning: a software program turned a knob, had a look at the result, turned some more, had another look, and so on, until individual cavity as well as the total machine voltage (i.e. energy) were at top performance and the whole system stable.
For the ILC, this means that not only the acceleration simulation models are already very good and can be improved still further with the new data, but also that operators know more about the range of knob-turning that might need to be done – and that they would like to have a few more knobs to turn. “It would for example be good to be able to adjust the relative power to each cavity,” explains Carwardine.
DESY’s FLASH accelerator itself had received a few more knobs before this round of ILC studies, and is now also equipped with a control mechanism that can ramp down radiofrequency power if cavities approached their quench limit, providing a soft upper limit to the cranking the 9-mA team was planning to do. The electron gun can now produce 800-microsecond bunch trains (operated this time at 4.5 mA) instead of the 400-microsecond ones it generated the year before.
“Every time we have come back to do our studies the machine has behaved and performed better,” says Carwardine. For their studies they rely on the support from the experts at DESY, who were not simply present during the studies, but heavily involved in them. So the international team was happy to be able to give something back, namely the possibility that FLASH could routinely reach higher voltages and higher machine energy for its intended purpose: operation as a free-electron laser. Already the photons produced for user operation are of just short enough wavelength to penetrate the so-called water window (when water becomes transparent), a property that is very interesting for studying biological and chemical samples at FLASH. Getting to higher linac energies would produce photons with shorter wavelengths and better penetration of the water window.
Another milestone result for the recent round of studies had to do with power rather than voltage, or gradient, limits. Understanding how close the ILC will be able to run to the power limit of the source of the microwave power – the klystrons – has been an equally important R&D goal, since klystron power is the second largest cost driver after voltage. The study required some clever re-working of the klystron electronics to mimic the ultimate high-power performance demanded by the ILC. The initial results indicated that the beam can be stably controlled with the klystron running within less than 10% of its maximum output. To put it another way, over 90% of the klystron power can be used to accelerate the beam. This means that the currently foreseen number of klystrons in the ILC is sufficient to accelerate up to 9 mA of beam to the required 250-GeV beam energy.
In September the 9-mA team will have another chance to push the limits – the last one in time to provide results and input into the ILC Technical Design Report. The primary goals will be to take what has been learnt and push to a 6-mA beam current at the highest possible beam energies – and all of that at the turn of a knob!
Recent Comments