The GDE design for the ILC as presented in the Reference Design Report (RDR) is for an electron-positron colliding beam facility having a maximum centre-of-mass energy of 500 gigaelectronvolts (GeV). No design or upgrade path to 1 teraelectronvolt (TeV) was presented in the RDR. However, the option to upgrade to higher energies was explicitly maintained in the reference design by ensuring that the beam delivery system could be upgraded, beam dumps were sufficient for higher energy and other considerations. Now, having had significant success achieving high gradients for the baseline machine and anticipating results that will guide the choice of energy from the LHC, we are undertaking a study of paths towards higher energy for the Technical Design Report (TDR) and are considering an R&D focus towards cost-effectively reaching higher energy for the post-TDR programme.
The GDE ILC design has been based upon the goals set out in an ILC Steering Committee (ILCSC) study entitled “Parameters for a Linear Collider,” authored by Sachio Komamiya and Dongchul Son (Asia), Rolf Heuer and Francois Richard (Europe), and Paul Grannis and Mark Oreglia (North America) with Rolf Heuer serving as chair. The initial report was written in 2003 and was updated in 2006. The 2006 report gave an energy upgrade the highest priority beyond the baseline for the ILC, as stated below:
3. Energy Upgrade beyond the Baseline machine
Independent of the results from the first few years of running there are several reasons for an energy upgrade. Examples include higher sensitivities for anomalous gauge boson couplings, measurement of the Higgs boson self coupling, the coupling of the Higgs to the top quark, production thresholds for new massive particles or exploration of extra spatial dimensions. Consequently, the energy of the machine has to be upgradeable.
The strong likelihood that there will be new physics in the 500 – 1000 GeV range means that the upgradeability of the LC to about 1 TeV is the highest priority step beyond the baseline.
- The energy of the machine should be upgradeable to approximately 1 TeV.
- The luminosity and reliability of the machine should allow the collection of order of 1 ab-1 (equivalent at 1 TeV) in about 3 to 4 years.
- The machine should have the capability for running at any energy value for continuum measurements and for threshold scans up to the maximum energy with the design luminosity (√s scaling assumed).
- Beam energy stability and accuracy should be as stated for the baseline machine.
For the new TDR baseline, most of the emphasis regarding energy has involved how to achieve the physics performance requirements at lower energies than 500 GeV. We have been very successful in developing a cost-effective and coherent TDR baseline that can meet the physics requirements, even at low energies. We are now embarking on a similar study of how to extend the energy reach of the ILC beyond 500 GeV cost-effectively and assessing the physics performance that can be achieved. This will give maximum flexibility in using guidance from LHC results for the machine that is proposed.
We are considering two approaches toward higher energy: 1) the option set out in the ILCSC study cited above of upgrading to 1 TeV at some future time, following initial 500 GeV running of several years; and 2) consideration of a more cost-effective direct path to a higher energy machine, especially if that becomes the priority from LHC results.
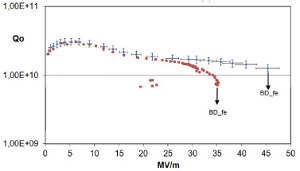
High gradients of 45 MV/m achieved with large grain nine-cell cavity at DESY (Aderhold et al)
The first approach involving a future upgrade to follow an initial 0.5-TeV ILC has always been part of the GDE mandate as set out in the ILCSC Parameter memos. The parameters and design of the 500-GeV machine uses today’s technologies, the enabling R&D is mostly in hand and we will be able to make a good cost estimate. We can extrapolate what upgrade technologies will be available some years after turn-on. We will be looking at the detailed questions of minimising what will need to be replaced from the initial 500-GeV machine, yet not be too constrained in the upgraded parameters by the initial machine. A specific plan for this option will be presented in the TDR, but the general plan will be to add higher gradient cryomodules to the end of the linac, plus other modifications that have been taken into account in the 500-GeV machine in anticipation of the upgrade.
The second approach is to design as cost-effective and as high-gradient a machine as possible for the initial collider, employing high-gradient cavities that will be tested in the post-TDR R&D programme, cost savings through value engineering and other technical innovations. Designing such a machine will put us in the most flexible position to adapt to whatever is learned from the LHC for the ILC construction proposal. We will use the TDR preparation period to define the post-TDR R&D programme aimed towards higher energy.
We are scheduling a special joint session with the experimentalists on higher-energy options at the LCWS11 meeting in Granada in September, and I will be periodically reporting on this programme as it unfolds.
[…] See the full and very interesting article here. […]