The popular understanding of a particle beam as a threadlike one-dimensional strand of energy isn’t wrong, but from the point of view of accelerator scientists, it’s a bit simplistic. Particle beams are nearly always carefully formed structures, especially in the space surrounding the point of their collision. Pinched here, stretched there, particle beams are crafted to squeeze the most luminosity and energy from their collisions.
Last month scientists decided on the best shape for an electron and a positron beam at the ILC with one teraelectronvolt (TeV) of centre-of-mass energy: its bunches are longer than initially designed and it has a shifted waist.
A particle beam’s waist, or focus, is the narrowest point along its length, where the particles that had been flowing merrily along are then packed into a tiny, compact space. To generate a healthy number of collisions, scientists guide the beams so that the densest parts of the oppositely directed beams meet up with each other, increasing the likelihood that their constituent particles will collide head-on.
The goal is straightforward. The execution isn’t.
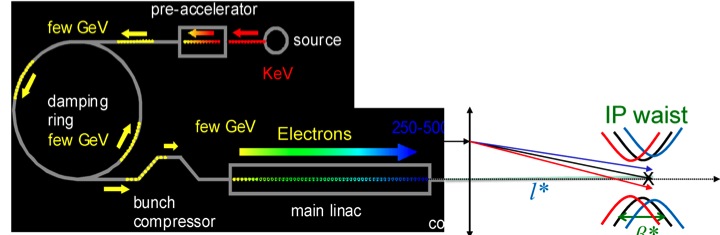
The red and blue lines represent a shifted waist away from the original focus, in black. ILC scientists chose the shift represented by the red curve.
Over the past several months, ILC researchers have been manipulating the length, placement and timing of the 1-TeV-beam waist, stretching or contracting it like a wad of taffy into different sizes and shapes by playing with various magnetic strengths and orientations to get the highest luminosity, or the most head-on collision, for ILC studies.
“It’s a physics-process-dependent balancing act,” said Jim Brau, research directorate contact for the Americas. The act involves balancing luminosity and background noise.
Through simulations, ILD and SiD detector scientists found a worrisome source of post-collision background interference, low-energy electron-positron pairs. These pairs would be kicked away from the beam at such an angle that they would strike the edge of the detector, multiplying into even more background tracks and clouding sought-after signals with their careless flights.
It is of course to a study’s advantage that, post-collision, the interesting particle signatures present themselves in the detector clearly, without unwanted noise obscuring events so hard-won by relentless and repeated shots at head-on collisions.
High luminosity is indeed hard-earned. To hit on the right waist placement for lots of collisions, ILC scientists considered several beam shapes, among them the travelling focus beam and the eventual winner, the shifted-waist beam.
In the shifted-waist scheme, the waist of both the electron and the positron beam would arrive not at the point of collision, which was the dynamic of the originally designed ILC beam, but just before the collision point. The shift of the beam’s narrowest point away from the collision site by just a couple hundred micrometres was shown to increase the luminosity by 10 to 15 percent over the original proposal. However, the shifted-waist shape couldn’t match the luminosity of its main competition in beam profile: its luminosity was about 5 to 10 percent less than that of the travelling focus scheme.
In the travelling focus scheme, one beam’s focus moves along with that of the oncoming beam, creating a strong, inseparable attraction between the two. Though this beam shape is shown to make wonderfully luminous collisions, it’s exceedingly difficult to achieve and requires devices that are still in the beginnings of the development stage.
CERN scientist Daniel Schulte suggested that the shifted-waist scheme could work for linear collider beams. Though shifted-waist has been understood for some time, it hasn’t yet been put into practice by any particle physics project. Now that the ILC project is receiving its finishing touches and its researchers have a firmer handle on how all the parts come together, it is at the stage where even modest luminosity gains of about 10 percent are worth pursuing.
Having milked the beam for that last bit of luminosity, the ILC team wanted to avoid undercutting this gain with too much background noise. This meant creating a beam shape that would help kick the noisy electron-positron pairs back down the beam pipe so as not to disturb the detector.
Last autumn, Kaoru Yokoya, Global Design Effort Asian regional director, proposed stretching the beam’s constituent particle bunches along their lengths and tightening their widths. He found that by lengthening the little bunches, he could reduce the kick-off angle of the renegade electron-positron pairs, redirecting them closer to the beam pipe and so away from the detector.
This bunch type carries a different risk – its longer shape amplifies the even the tiniest inadvertent initial up- or down-misplacement of the beam, leaving little room for error in the beam setup. Last month researchers agreed to a bunch length of 225 micrometres, finding a nice compromise between reduced background-pair angles and safe initial setup.
“Now it’s possible to keep this very high luminosity but to calm the pairs down to a smaller cone that’s compatible with the design of the detectors at 500 gigaelectronvolts,” Brau said.
The ILC is fully designed for 500 GeV of collision energy, but the machine will also be able to be upgraded to deliver twice that, one TeV of energy. In designing a suitable beam with double the energy of the baseline design, scientists had to come up with a beam that the detectors, designed for the 500-GeV collider, could handle. Now that this latest step in the 1-TeV upgrade is finalised, researchers will likely adopt a similar beam profile for the 500-GeV technical design as well.
The beam specifications will be documented in the 2013 Detailed Baseline Design and the Technical Design Report.
Leah is to be complimented on a very clear presentation of a difficult technical subject!