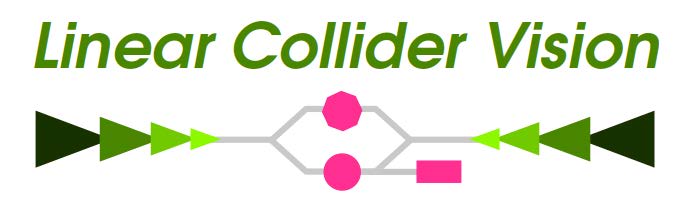
The previous iteration of the European Strategy for Particle Physics (ESPP) gave as its first recommendation: “An electron-positron Higgs factory is the highest-priority next collider.” This recommendation is, in our view, best addressed by constructing an e+e− Higgs factory that meets all of the physics goals of such a collider in a way that is affordable for the global particle physics community and can be realistically implemented in the shortest possible timescale. With a solution based on an e+e− linear collider, all of these criteria can be met — while at the same time preserving the potential to react to results expected from the High-Luminosity LHC and other developments in global particle physics.
There is a reason why the study of the Higgs boson in e+e− reactions is considered the most urgent problem in particle physics. We have seen in experiments over the past three decades that the Standard Model provides an excellent description of the fundamental forces acting among elementary particles. This success highlights a set of problems in particle physics that the Standard Model is incapable of answering: What determines the spectrum of quark and lepton masses and mixings? Why is CP violated? What is the origin of the neutrino masses? In the Standard Model, all of these effects are due to the vacuum expectation value of the Higgs field, yet we have no understanding of why the Higgs field obtains a vacuum value in the first place. If these questions have explanations, they must come from new physics beyond the Standard Model that couples to the Higgs boson. Thus, the search for beyond-Standard-Model effects on the Higgs boson, using the highest precision that we can muster, is our best chance to discover new fundamental interactions beyond those currently known.
An e+e− collider offers many observables that can be sensitive to beyond-Standard Model effects on the Higgs boson. First, there are the Higgs boson couplings giving decays to the fermions and bosons of the Standard Model, and also to unexpected final states, for example, through the Higgs portal to new sectors. In addition, there is the Higgs self-coupling, most robustly measured in HH production, the process of creating two Higgs particles. Finally, in models in which the Higgs boson is composite, the top quark must couple to the new compositeness interactions, and these become visible in the top quark Yukawa coupling, in the top quark electroweak couplings, and in top quark 4-fermion interactions. All of these observables can be explored by e+e− measurements, which will significantly improve upon the precisions available from the LHC. Measuring all of the observables, however, requires e+e− centre-of-mass energies up to 1 TeV.
It is important also to understand that the discovery of new interactions through precision measurements has a very substantial burden of proof. To convince the particle physics community, it is not enough to have one highly significant measurement of a deviation from the Standard Model. We will need complementary measurements of these effects, in reactions with different sources of systematic uncertainty. Fortunately, a complete study of the Higgs boson in e+e− reactions provides this. A deviation in Higgs couplings obtained in the reaction e+e− → ZH can be confirmed in Higgs production by WW fusion. Similarly, an effect in the Higgs self-coupling measured in e+e− → ZHH can be confirmed with a measurement in WW → HH. The natural evolution of the physics of e+e− reactions with centre-of-mass energy gives many more examples. For this also, a large range of centre-of-mass energies must be studied.
In earlier ESPP updates both ILC in Japan and CLIC at CERN have submitted strong project proposals. These two projects will also be presented to the ESPP this time, including their latest parameters and technical developments. However, another very interesting and novel implementation path is possible for a linear collider, combining the unique industrial readiness and large linac project experience with Superconducting RF (SCRF) technology as proposed for ILC, with the upgrade possibilities offered by high gradient technologies as used in CLIC, C3 and others.
Such a program can be implemented quickly with modest technology and financial risk, and remains very ambitious in its scientific goals and reach, including several possible paths for energy and luminosity upgrades. The remit for the ESPP encourages ambitious proposals for the next flagship project at CERN. CERN, after all, has constructed and operated the LHC, so it has the experience and capabilities needed to lead a new project of similar magnitude and technological breadth.
The LC Vision initiative thus proposes an ambitious Linear Collider Facility to be constructed at CERN. It will be executed in stages, where the first stage is an e+e− linear collider based on SCRF technology. SCRF is widely available today and would allow the fastest realisation of a new facility. Depending on the level of external contributions beyond the CERN budget that were to be acquired for the realisation of such a project, it could start at a centre-of-mass energy of 250, 380 or 550 GeV, with, respectively, tunnels of 21 km, 27 km, or 33 km in length. Likewise, the luminosity of such a machine can be staged depending on the resources, potentially offering four times the luminosity of the initial stage of the ILC as proposed for Japan. A rigorous costing of such a proposal is being prepared for the ESPP. After the initial technology has been fully exploited, the Linear Collider Facility would be upgraded in energy and other capabilities by introducing new technologies. This could be advanced SCRF with gradients of 40–45 MegaVolts per metre (MV/m), but several technologies capable of producing accelerating gradients of 70 MV/m and above are under study. These have very different levels of maturity but all target implementation readiness during the next decade. These are the CLIC two-beam accelerator mentioned above, the C3 cold copper accelerator, and the HELEN advanced superconducting RF accelerator. With 70 MeV/m in a 21-km tunnel, the collider would reach centre-of-mass energies of at least 1 TeV, in a 33-km tunnel 2–3 TeV.
At a centre-of-mass energy of 250 GeV, such a machine can efficiently record 4 ab−1 of data, roughly 1 million Higgs bosons, each tagged by recoil against a Z boson. Using beam polarization, the precision on Higgs couplings is similar to that obtained with 10 ab−1 at an unpolarized collider. This stage would also include a two-year run at the Z0 pole, achieving a precision measurement of sin2 θw comparable to that expected from the FCC-ee TeraZ programme. The programme would be implemented with two interaction regions sharing the total luminosity.
At 550 GeV, an additional 4 ab−1 could be recorded, with, also, 200 fb^{-1} at the top quark pair production threshold. Then — noting that the luminosity of a linear collider increases linearly with the centre-of-mass energy — a further 8 ab−1 of data could be recorded at 1 TeV or beyond. In this regime, Higgs production is dominated by WW fusion, giving the independent precision measurements of Higgs couplings discussed earlier. Running at two distinct energies well above the top quark threshold also allows a complete exploration of the top quark couplings. Finally, this programme offers measurements of Higgs pair production in the two complementary reactions described above. All of these measurements are beyond the capabilities of circular Higgs factory colliders.
This proposal makes good sense for CERN. Any large project at CERN would require a preparatory phase of about five years and a construction period of about ten years. Starting today, this brings us to the last years planned for the operation of the HL-LHC. Starting the next programme within a short time after the end of the HL-LHC will allow young scientists to participate in the design and construction of the new detectors while gaining experience with data-taking and analysis on an operating collider. This will ensure that the next generation of particle physicists can acquire all of the skills that they will need to carry our field forward. Significant delays from this schedule would diminish our future capabilities.
Regarding the even farther future, particle physicists look forward to exploration of parton-parton or lepton-lepton centre-of-mass energies ten times greater than those available from the LHC. Today, we do not have a technology that can achieve this goal at reasonable cost. A proton-proton collider will always have the disadvantage that its very large footprint will require an expense many times that of the LHC, a level of cost that is unaffordable today, and remains a serious uncertainty and concern also in the future. New particle acceleration technologies — for example, the muon collider and plasma wakefield acceleration — are now under study. We expect that, in the next 20 years, fundamentally new designs for high energy colliders will give us a robust route forward.
Our proposal will encourage this route on the side of electron colliders and accelerator R&D, offering opportunities to extract a small fraction of the bunches for tests of new technologies. Possibilities for our linear collider facility include the demonstration of photon collider technology, energy recovery for dramatically increased luminosity, and a plasma wakefield “afterburner” to raise the energy of an e+e− collider.
We strongly believe that this novel Linear Collider Facility proposal is one that CERN needs to consider very carefully now.
- It is rooted in the need for precision studies of the Higgs boson as the next major goal in particle physics, while preserving at the same time also the potential to react to results from the HL-LHC.
- It can be constructed on a time scale appropriate for the successor to the HL-LHC.
- It is affordable for our community, with the possibility of designing a first stage to be consistent with the current CERN budget and an evolution that can also be funded within expectations.
- With global support, this project is compatible with the increased accelerator R&D, along multiple routes, that we will need to propose a 10 TeV parton or lepton centre-of-mass collider in the foreseeable future.
- And, our proposal itself incorporates technological innovation that will benefit many stakeholders outside of particle physics.
A set of documents laying out this proposal in detail is now being prepared by the LC Vision initiative for the ESPP. We encourage everyone to read these and to provide their support by signing here. The author thanks Masaya Ishino, Jenny List, Tatsuya Nakada, Roman Poeschl, Aidan Robson, Steinar Stapnes, and the participants in the LC Vision Community Meeting for many fruitful discussions to develop the ideas presented here, and for important comments and suggestions on this report.
Thanks Michael ! I hope this proposal also makes it into the upcoming European Strategy report…